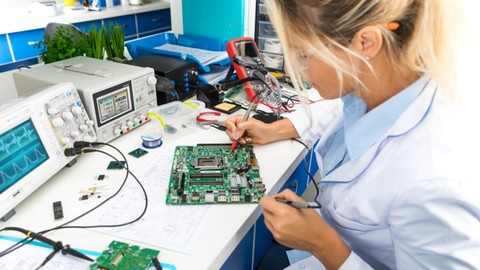
Electrical Engineering Full Blueprint – 200 Hours HD Video
-
More than 300 Topics -
Check the lectures
- begginers are welcome
So, we want to send the power from the source to the load. So, here you can see that we have a source here and we have a load here. We are assuming the load to be a resistive load here. So, if we connect or if we analyze this circuit, this is a DC source and a load. We see that energy is flowing from the source to the load, but there is no control of the flow of energy ok. So, it is a fixed source and a fixed load and we cannot control the flow of power from the source to the load.
So, how can we control the power flow? The easiest way of doing it is to add a potential divider or a resistive voltage divider as it is shown in the second figure, the resistive voltage divider here. Now, if I add the resistive voltage divider and by varying this resistance on the variable resistance, I can control the flow of power into the load. However, although this system will function, however, this is not an efficient system because when we transfer the power through the voltage divider or through the potential divider or through this resistive voltage divider circuit, we lose a substantial amount of power. In particular, when we talk about high power levels is not at all suitable. This circuit is not at all suitable because it will cause a large amount of heat dissipation in this particular resistive voltage divider circuit and subsequently the efficiency of the system will go down. In all this high power conversion, efficiency is a very important factor and there are two reasons for that. The first reason why the efficiency is important is if you do not have the high efficiency you lose the energy.
So, that is one important factor for any system that you want to whatever is the desirable power flow, you want to do it with the maximum efficiency so that you get the maximum energy from the input, but there is another very important reason why efficiency should be very high because if efficiency is not high, then the losses in the system is very high. So, if the loss in the system is very high, then it appears as heat in the system which we need to dissipate. You must have seen power converters with heat sinks typically aluminum heat sinks are very frequently, we have seen. Heat sinks constitute a major component in all power converters because it occupies a substantial volume and also a weight of the converter. So, if the efficiency is low, that means, the losses in the system is high which in turn means that the high size of the heat sink should be bigger because we have to get the heat out of the system. So, let us take the example of power converter which is handling about 1 megawatt of power. Suppose there are two converters and the first converter runs at an efficiency of 99 percent and the second converter runs at an efficiency of 95 percent. Now in the first converter the losses in the system is 1 percent of 1 megawatt and which is equal to 10 kilowatts and for the second converter, the losses are 5 percent. So, the loss is equal to 50 kilowatts.
Now this means that all this loss is appearing as heat in the power converter and which must be totally dissipated into the ambient either through by natural cooling by air or by forced cooling through water or some kind of oil for example. So, the losses play a very vital role in the power conversion process and there is also one principle which is usually followed in the industry. This is the Arrhenius principle and it says that every 10 degree centigrade rise in the temperature of say a power device will reduce its lifetime by half. This is very important because if you operate a power device at an elevated temperature for prolonged duration of time, you are reducing the lifetime of the converter. So, keeping the temperature under control in power devices or power converters is very important and there are lot of techniques to do that. Coming back to what we were discussing. So, here we see that we have a source and we have a load and the circuit although there is a potential divider or a resistive voltage divider, but unfortunately this is not a very efficient circuit because a lot of heat will be dissipated in the potential divider. (Refer Slide Time: 08:35) So, what is the solution for this? The solution for this is to use a switch which I have shown here, this is the switch here. Now when you connect the switch then suppose this is an ideal switch I mean it does not dissipate any heat. So, and it is a fully controllable switch which means I can turn it on and I can turn it off with control signals which is totally controlled by myself or by me. So, then we see that with this switch power flow to the load can be controlled by turning on and off this switch. If I turn on the switch, then the voltage of the source is impressed across the load. If I turn off the switch, no voltage comes across the load.
So, the voltage on the load is a series of pulses which is shown in this diagram. So, when I turn on the switch that is this T on period, then the load voltage is equal to the source voltage. When I turn off the switch the load voltage is 0. So, by controlling so, therefore, there this Vload which I have shown here the expression of Vload is So, therefore, we see that the load voltage can be changed by changing the duration of Ton over this Ton plus Toff time, Ton plus Toff time is sometimes called the switching period. So, we can change the magnitude of the load voltage. So, we can use a switch to decrease the losses and increase the efficiency of power conversion from the source to the load. (Refer Slide Time: 11:25) Now, although this arrangement of load and source with the switch works well for resistive loads, unfortunately most of the power electronic converters have inductive loads that is R-L loads, resistive inductive load or very pure purely inductive loads or highly inductive loads. So, often powered electronic converters feed to loads for example, motors, transformers etc, they are inductive in nature. Now once the load becomes inductive in nature, we face a challenge and that challenge is because of the property of inductive loads. Inductive loads are represented as current sources in the circuit ok.
Now current source when you put into such a circuit along with a switch, then we face a challenge. (Refer Slide Time: 12:29) What is the challenge? The challenge comes from the property of current source. You know current source and voltage source they are dual in nature in terms of their properties. For example, current sources can be shorted, but they cannot be opened. On the other hand, voltage sources can be opened, but they cannot be shorted. For example, if you take a voltage source say a battery and then you can keep it open, you can take the battery in your hand and move round nothing will happen because you have opened the battery or the voltage source. But if you short-circuit the terminals of the battery, then a very large current flows through the wire which is shorting the battery. If the voltage source is an ideal voltage source then the current which is flowing during this short circuit will be enormously high, it will be ideally infinite. Practically it will be very high. So, we cannot shortcircuit a voltage source because the current will be enormously high or we do not know: what is the exact magnitude of the current only limited by the internal impedance of the voltage source. Similarly, for a current source, we can readily short-circuit a current source, but we cannot open it because if we open a current source, then we do not know the voltage across the current source how much voltage will come across the current source, it will be very high. So, in this circuit, this current source here also we have to follow for this current source the same principle that it can be shorted, but it cannot be opened. So now, therefore, in order to satisfy the property of both the voltage source and the current source, we need two switches ok, switch S1 here and switch S2 here. Basically we are transferring power from the voltage source to the current source using two switches here. So, what are the functions of these two switches? Switch S1 opens the voltage source and it isolates the voltage source from the circuit whereas, switch S2 short circuits the current source ok.
So, switch S2 here short circuits the current source and switch S1 here, opens the voltage source. So, we have achieved the first criteria of voltage source and current source. (Refer Slide Time: 15:59) Note that these two switches S1 and S2 cannot be operated together. For example, if I turn on both S1 and S2 together at the same time, then I am going to short circuit the voltage source, which is not allowed. In a similar way, I cannot open both these switches together. At the same time in that case, I will open the current source which is again not allowed. So, therefore, these two switches S1 and S2 must be operated in a complementary fashion like when S1 is on, S2 is off when S2 is on, S1 is off like that. So, they must be operated in the complementary fashion. So, how do we realize these switches in the real life power devices? (Refer Slide Time: 17:05) There are many ways to realize these two switches ok. One way is to use for example, IGBTs or MOSFETs along with diodes. This is one of the simplest way of realizing these switches. For example, you can see on this circuit we have realized using IGBT and diode. Switch S1 here is replaced by an IGBT switch S2 is replaced by the diode. In this lower circuit, you can see switch S1 is replaced by the MOSFET switch S2 is replaced by the diode and we have the voltage source on the left and the current source on the right.
This is one way of realizing this circuit using IGBT or MOSFET. We can also use other types of switches; for example, thyristors can be used. However, in this course, we will not talk much about thyristors. The reason is many past decades thyristors have been quite popular. But seeing the future we see that in many of the applications thyristors are going to be replaced or have been replaced with IGBTs or MOSFETs because one of the reasons is thyristors can be turned on, it is a semi controlled device. It can be turned on, but it cannot be turned off, the circuit decides how to turn it off. So, we see that thyristors are going to be replaced mostly with IGBTs at the medium voltage level and at the low voltage level MOSFETs will be predominant that is what we see in near future say for at least 10 to 15 years from now. So, we are not going to talk about thyristors much in this course.
Of course, thyristors will be used at very high power levels in LCC based HVDC for example. We will still continue to use thyristors, but they are very specific applications and in many new developments that is going to that is going on here now in the field of high-power high voltage HVDC for example, power electronics newer developments are all happening with IGBT. So, and in particular, we can also say that with wide band gap devices coming at least at the lower voltage levels huge presence of wide band gap devices are expected in near future. So, we will not talk much about thyristors in this course and mostly concentrate on IGBTs and the medium voltage level and sometimes MOSFET at the low voltage level. (Refer Slide Time: 20:41) So, let us take the case of this converter with an IGBT based realization which I have shown in this circuit here. We have a voltage source, we have the current source, the IGBT and the diode here. Now what is a switch rating? Switch rating is the rating of the switch that we are going to use. There are two there are many ratings of the switch which you can see from the datasheet of a switch, but there are two very important rating of a switch. One is the voltage rating and one is the current rating. What is a voltage rating of a switch? The voltage rating of the switch is defined as the voltage across the switch when it is off. So, when the switch is off how much voltage is impressed across the switch that determines the voltage rating of the switch. What is the current rating of the switch? The current rating of the switch is the current flowing through the switch when it is turned on. There are many other ratings of the switch for example, temperature rating of the switch and then there is also transient rating of the switch. Many other rating of the switch is present which if you open the datasheet of any device, you can see the different ratings of the switch. But these two rating are very important voltage and current rating of the switch. So, let us try to find out first what is the voltage and current rating of the IGBT and diode in this particular circuit. So, we want to find out the voltage rating of IGBT. So, in order to find out the voltage rating of IGBT, we see or we try to find out what is the voltage across the IGBT when it is turned off. So, when it is turned off the circuit when the IGBT is turned off, the circuit looks like this.
As I have told earlier that S1 and S2 are complementary in nature. So, if the IGBT is turned off the diode is turned on so, I have put a short circuit here and through this short circuit the load current is flowing. Since the diode is short circuited therefore, the negative terminal of this source is connected here while the positive terminal is connected here. And so, we can see that the voltage across IGBT during this time is equal to the source voltage. Now what is the current rating of the IGBT? The current rating of the IGBT will be equal to the load current. Because when the IGBT is conducting, the diode will be turned off because it will be reverse biased and so, the current of the load current is flowing through the IGBT and therefore, the current rating of the IGBT is equal to the load current. So, the voltage rating of the IGBT is equal to the source voltage and the current rating of the IGBT is equal to the load current. What about the diode? (Refer Slide Time: 24:41) Again for the diode, we see that when the IGBT is turned on, then the diode is turned off. And since the diode is turned off and the IGBT is turned on so, this terminal positive terminal comes here and the negative terminal is here. So, the voltage across the diode is equal to the source voltage. So, therefore, voltage rating of the diode is equal to the source voltage and what is the current rating of the diode? The current rating of the diode I have already shown it here is equal to the load current. So, therefore, the voltage and current rating of both the IGBT and the diode are same. Voltage rating is the source voltage and current rating is the load current. (Refer Slide Time: 25:47)
Now, the circuit which we have so far developed is this one with the switch, diode and the voltage and current source. Those who are a little bit familiar with power electronics will immediately see a similarity of this circuit with a buck converter. I have drawn a buck converter here and the buck converter also has a voltage source and then, there is a load, there are two switches here. There is an inductor and a capacitor. If we consider the current flowing through the inductor as a current source, then we see that the buck converter here has very much similarity with the circuit that we have developed so far. So, I can also redraw this circuit whatever I have drawn, I can draw it a little bit differently the same circuit, but I have drawn it a slightly different as I am showing it here in this circuit. So, I have placed the switch in a vertical position and the diode here at the bottom and the current source like this. This circuit is exactly similar to the left hand circuit. So, both these circuits are same, I have just redrawn it. I will come back to this circuit a little bit later. (Refer Slide Time: 27:43) Now, suppose I have changed the direction of the current. So, now, here let us assume that we have changed the current direction from right to left. Earlier it was from left to right, now I have changed the direction from right to left ok. What will happen now to the circuit? So, how can I realize the circuit now? So, what I can do? This is one way of realizing the circuit as you can see here, I have interchanged the positions of the diode and the transistor. So, the IGBT has come down here and the diode has been moved here compared to the previous circuit. So, this is one way of realizing a circuit with the voltage source here and the current source here where the current source the current direction is from right to left. (Refer Slide Time: 29:01) So, this circuit where the current direction of is from right to left is very similar to a boost converter, but you have to look from right to left. So, for example, this is a traditional boost converter and you have this is the voltage source and this is the load here. In a boost converter, we have an inductor at the front part of the circuit and then we have two switches here and then the capacitor which acts as a voltage source. So, if we replace this inductor with a current source like this, then we see that looking from left to right looking from in this direction from left to right, we see that there is a current source and there is a switch controlled switch and there is a diode here and then there is a voltage source here; the capacitor is a voltage source here. Similarly looking from on this circuit; if we look at in this circuit and see that there is a current source here, there is a switch here, there is a diode here and there is a voltage source here. So, for this circuit looking from right to left and for this boost converter looking from left to right, we see that these two circuits are very very similar ok. Looking from left to right, there is a current source, switch, diode and voltage source, looking from right to left current source two switches and a voltage source here. So, they are very similar.
Again therefore, what I will do now I will again redraw this circuit in a slightly different fashion as I have shown it here. You can see the voltage source is here and the voltage source is here. The current source is here and the current source is here. The switch the transistor here S2 has been now put down here and the diode there has been redrawn in a slightly different fashion here. (Refer Slide Time: 31:55) So, we have now redrawn the circuit. Now, combining this circuit which was derived from the buck converter analogy and the circuit which was derived from the boost converter analogy, we can combine these two circuits. And how does it look? It looks like this. This is the current source present which is shown by an R-L load here, there is the current source. The current source is bi-directional in nature. So, it can be positive or negative. This is the voltage source here and then we have two transistors or two IGBTs here and two diodes here. So, we see that from the buck converter and boost converter topologies, the analogy from that analogy we can combine this buck and boost converter with a single circuit like this and this circuit is called the half bridge converter. The half bridge converter is one of the basic topologies of our electronics. And also for this course half bridge converter is something which will be used frequently and derivations of half bridge. So, understanding the half bridge converter is very important for this course. So, we see that in this half bridge circuit, the voltage here at point A can be controlled by the switches S1 and S2. If we measure the voltage at point A with respect to point O at the bottom, then we see that the voltage at point A can be either 0 or VD. So, when the lower switch is on then the voltage is 0 between A and O and when the upper switch is on the voltage is VD. So, the voltage between point A and O is unidirectional, however the current which is flowing through the load can be bi-directional. So, it can be both the current can be both from left to right or from right to left that is both positive and negative current is possible. And depending on the voltage and the current situation at any instant of time, all four switches here that is the two IGBTs and the two diodes, they will be conducting depending upon the situation. So, let us see how this happens. (Refer Slide Time: 35:41) Say for example, if the voltage VAO is equal to VD, now if the switch is S1 and D1 either of them if the upper switch is on, then the voltage V is VD. When the lower switch is on, the voltage is 0. When the upper switch is on either S1 or D1 will be conducting when the lower switch is on either S2 or D2 will be conducting.
So, if S1 gate signal has been given then the upper switch turns on the voltage between point A and O will be like this here, suppose the current waveform is alternating in nature. So, sometimes the current is positive and sometimes the current is negative. Let us take the interval t1 where the current is positive while the voltage between points A and O is 0, which means the lower switch is on. During this time, we have now two possibilities either S2 will be on or D2 will be on and we see that because the current is positive direction, that means the current is flowing from left to right only D2 is conducting, because D2 has this path and the path of the current is like this. So, the path of the current is shown here this is the path of the current and D2 is conducting so, the circuit is getting completed like this D2 iload and like this here. So, we see that inspite of giving pulse to S2 during this time, the IGBT is not conducting it is the diode D2 which is conducting and the voltage between point A and O is 0, because if it is an ideal diode, the voltage drop across the diode is 0. So, basically point A and O are at the same potential. (Refer Slide Time: 03:09) Now, let us take the second interval t2 here. During the time interval t2 we see that we have given gate pulse to S1 while the current is still positive, if this is the case then we see that we have given the gate signal to S1 so which means either S1 or D1 will be on and since the current direction is positive therefore, the IGBT here must conduct because this is the path of the current. So, it is flowing through the IGBT, through this here, through current source and then through the voltage source and there is the path complete. So, during this time interval t2 we see that the voltage between point A and O because switch S1 is conducting the voltage between point
A and O will be equal to VD. (Refer Slide Time: 04:27) Next let us see the case during time interval t3. So, we are looking now into this time interval t3. During this time, we see that the gate signal has been given to switch S1 while the current is negative. So, since gate signal has been given to S1 so, the either S1 or D 1 will be conducting here. In this case since the current direction is negative that means the current is now flowing from right to left here. The current must now flow through diode D1 here and when diode D1 is conducting assuming an ideal diode the potential at this point and at this point becomes same and hence the voltage at point A with respect to point O will be equal to VD. So, we get a positive voltage. So, VAO here is a positive voltage the diode D1 is conducting and the current is negative here. (Refer Slide Time: 06:03) Let us take the last case, where the gate signal has been given to the switch S2 while the current is flowing negative. In this case we find that switch S2 is conducting here. So, we have analyzed the 4 switches of the half bridge converter and when they will be conducting at different time instants. In summary we can say that the voltage that is VAO produced by the half bridge converter can be unidirectional in nature while the current can be bidirectional in nature. Before I go to full bridge converter I must stop here for a minute and explain something which needs some attention. (Refer Slide Time: 07:33) So, if you see here, we have so far talked about a circuit like this where we have said that this is point A and this is point O, this is the circuit which we have talked about. Often we represent this circuit as something like this, 2 ideal bi-directional switches. Now, in many literatures and also in books you will find an alternate representation. What is the alternate representation? In the alternate representation we have a circuit something like this. VAO like this, what is the difference? First of all, understand that in this circuit the point O is actually an imaginary point, it is a fictitious point. In a real circuit say for example, you analyze a half bridge, how will you get the DC bus of the half bridge? You will do it from a rectifier. For example, the rectifier circuit, say if you take a 3 phase rectifier, here comes the DC bus.
The DC bus is made up of capacitors and there is no midpoint of these capacitors unless someone makes a special connection to get a midpoint of the capacitors. Normally, it is just a capacitor there is no midpoint; however, in many books and literatures also you will find that the O point has been taken here. Now, this means there is a reason for it, remember this O point is an imaginary point it does not exist in a real physical system. (Refer Slide Time: 11:07) Now, if you analyze the 2 circuits for example. Suppose, we analyze these 2 circuits then we see that here the VAO voltage is always fluctuating between 0 and VD, where VD is this voltage here. The VAO voltage always oscillates or fluctuates between 0 and VD. On the other hand, the VAO voltage here always fluctuates between VD by 2 or minus VD by 2. So, we see that in this case the when they say for example, in you have S1 and S2 here and S1 and S2 here. If S1 is on in this circuit here if S1 is on then VAO is VD by 2, if S2 is on VAO is minus VD by 2. On the other hand, here if in VAO, it is 0 or VD. So, we see that if we use this circuit then there is always a DC in VAO voltage produced, because it is always oscillating between 0 and VD whereas, in this circuit we always oscillate between plus VD by 2 and minus VD by 2. These two circuits are equivalent only the difference is that in this circuit there is always a DC offset in VAO waveform. So, the presence of this DC offset or the DC offset if we can remove it by using this circuit, then the analysis becomes much simpler and so, many people prefer to use this circuit here with O point at the middle. Many people prefer this circuit to be analyzed because the DC offset in VAO voltage does not exist in this circuit, but this circuit analysis is more close to the real system. So, therefore, we will use we can use either of them, but in the present analysis we will use this circuit here all the time, because this is more real this is closer to the real system. So, this VAO is between 0 and VD this is what we will assume. However, remember that this analysis with plus VD by 2 and minus VD by 2 is also equally valid. (Refer Slide Time: 15:03) Now, so far we have analyzed the half bridge circuit. Now, let us add one more leg to this converter which is called in fact, the full bridge converter. So, here you see that, this is one half bridge and we have added one more half bridge to the circuit.
Note that these 2 half bridges are completely independent of each other. In fact, they do not know their existence, they are just operating independently. So, the left hand half bridge does not know about the right hand half bridge. So, here why are we then adding this second half bridge? The second half bridge is added mainly because we want to get bi directional voltage across the load. Remember in the half bridge with a single half bridge we got unidirectional voltage and bi-directional current. Now, with the full bridge it is possible to have bi directional voltage across the load, but more importantly there is another advantage. The advantage is that we get double the voltage from the same DC link using the full bridge as compared to the half bridge. So, first let us understand how the full bridge is operating. (Refer Slide Time: 16:59) So, in the full bridge of course, as I told you there are 2 half bridges which are operating independently of each other. So, if you want to get so, there is now this leg, there is point A here and there is point B here. So, if we want to get the VD voltage here then you can turn on S1 and S4. If you turn on S1 and S4 then if you turn on S1 then A point is shorted to the positive terminal, if you turn on S4 B point is shorted to the negative terminal and so, the voltage VAB is equal to the DC bus voltage. As you can see here, if this is turned on so which means that this point is shorted to this point and this point is shorted to this point. If I turn on S1 and S4 the diagonal switches if they are turned on so, I can see that the voltage VAB is nothing, but VD. Similarly, if I turn on the other 2 diagonal switches and these other 2 diagonal switches are say S3 and S2 here, then I can get minus VD across the load.
On the other hand, if I turn on both the upper switches or both the lower switches together then I get a 0 voltage across AB, because if I stop suppose I turn on the lower 2 switches then A point is shorted here, B point is shorted here and I get a 0 voltage here, basically the load is freewheeling during that time, it also happens when you turn on both the upper switches is like this here. Now, similar to half bridge where we analyzed the operation of all the 4 switches, I mean 2 diodes and 2 transistors here also we can analyze the operation of all the 8 switches here. (Refer Slide Time: 19:43) I will not go into that details, but I will only analyze 1 or 2 of them. For example, so there is a VAO voltage which is VD and then there is a VBO voltage which is shown here and it depends on how we have applied the gate signals. In this case the gate signal S1 and S4 is applied. So, VAO voltage becomes VD here and gate signal 2 if you apply here it becomes VBO like this. So, during this time what is the VAB voltage, VAB voltage is nothing, but VAO minus VBO. VAB=VAO-VBO So, I can see that the, if I subtract VAO minus VBO in these 2 curves I see that VAB voltage fluctuates between plus VD and minus VD here. Now, the current can be again depending on the power factor, the current can be sometimes positive and sometimes negative with respect to the V AB voltage. So, let us take the time duration t1 where the VAB voltage is minus VD and the current is positive and so, I can find out which are the switches conducting. So, first I will see that I have applied the gate signal to S2 and S3. So, these two diagonal switches must be conducting at this point. Now out of them which one is conducting, whether the diode is conducting or that IGBT is conducting that can be understood from the direction of the current. So, here the current direction is positive so, which means the current is flowing like this. So, it must flow like what is shown by the dotted line here, diode, the current and through this diode D3 and then comes back.
So, this must be the path of the current here what is the voltage. So, VAB voltage so, because the diode D2 is conducting. So, O point is shorted to A and D3 is conducting. So, B point is shorted to the positive terminal and therefore, the voltage of minus VD is getting applied across VAB here. (Refer Slide Time: 22:55) In a similar way you can also do the analysis for other durations. For example, if you take the t4 duration you can make a similar analysis and can find out that the switches S3 and S2 are conducting just like how we have done with a half bridge. So, in this fashion it is possible for us to know all the 8 switches that 4 transistors and 4 diodes how they are conducting in this full bridge converter, ok. But, remember that one advantage of using a full bridge is that in a half bridge remember that the load voltage was fluctuating between 0 and VD whereas, in this case in a full bridge converter the load voltage is fluctuating between minus VD to plus VD, which means that in a full bridge converter 2 half bridges are working together and we can make them work in such a way that we get double the voltage out from a full bridge converter as compared to the half bridge converter, ok. So, this is the other advantage of a full bridge converter as compared to a half bridge converter, although we are using more number of switches, but we are getting double the voltage across the load, ok. (Refer Slide Time: 24:45) Now, before the next step in our analysis we will see what is called as a pulse width modulation. Why do we use pulse width modulation, because we want to control the voltage magnitude across the load. If you see here in for example, the full bridge the voltage magnitude across the load is always fluctuating between minus VD and plus VD and we cannot control the output voltage magnitude. So, by introducing notches in this voltage waveform which we will do using pulse width modulation, we will be able to control the output voltage magnitude. So, let us see how we can do it. So, what we will do is, we will turn on and off these switches S1 and S 2 repeatedly by following a specific pattern. (Refer Slide Time: 25:57) So, let us see how we can develop this pattern.
So, in this pulse width modulation technique the output voltage is controlled by a series of pulses of voltage. So, if the pulse width is more, more is the average voltage across the load or more is the output voltage of the converter. So, in this technique there is one high frequency triangular wave. So, this is the triangular wave which is part of the triangular wave is shown here basically the triangular wave exists like this, ok. So, this is the high frequency triangular wave and we also have this as the modulating wave or the reference wave. So, there is a high frequency carrier wave or the triangular wave and it is compared with a low frequency modulating wave or a reference wave. The low frequency reference wave can also be a DC for example, in this diagram you can see that the modulating waveform here is not changing over the time interval which we are considering here. So, the red one is the modulating waveform here, it is value is m. The triangular wave is spanning between 0 and 1 as you can see here this is the 1 and there is the 0, the triangular wave or the high frequency carrier wave is spanning between 0 and 1 and the modulating wave at this instant of time is somewhere in between and it is amplitude is m, ok. (Refer Slide Time: 28:05) Now, we in order to control the output voltage of this half bridge we follow a logic. What is the logic?
The logic is if the modulating wave is more than the triangular wave then I turn on S1 otherwise I turn on S2. So, let us apply this logic and see how the output voltage of the converter can be changed. So, here is the triangular wave and here is the modulating wave. So, if the modulating wave is greater than the triangular wave I will turn on S1. So, which means that during this portion of time, the modulating wave is more than the triangular wave. And hence I will turn on S1 in this circuit I will turn on S1, as I turn on S1 the VAO voltage will be equal to VD that is the dc bus voltage. The rest of the time for example, here or here the modulating waveform is less than the triangular waveform and so, I will turn on S2 that is the lower switch and therefore, I will get a 0 voltage here and here. So, you can now see that by changing the height of the modulating waveform in comparison with the triangular waveform height I can change the width of this pulse which is coming across VAO. So, if the modulating waveform is lower, for example if the modulating waveform is somewhere here then I will get a very narrow pulse. So, if the modulating waveform is somewhere here then I will get a very narrow voltage waveform here or a narrow pulse and you can understand that the average VAO will be low in magnitude. On the other hand, if the modulating waveform is high with respect to the triangular waveform I will get a big pulse like this. So, therefore, by changing the position of the modulating waveform in comparison with the triangular waveform I can change the average output voltage VAO. The instantaneous output voltage VAO is always fluctuating between 0 and VD. However, the average output voltage of VAO can be changed by changing the position of the modulating waveform with in comparison with the triangular waveform. So, what is the average voltage of VAO during the switching cycle TS? So, when we say about average voltage we must specify a time period that is the time period in which we are going to do the averaging. (Refer Slide Time: 31:51)
So, this time period in this case we are assuming the time period of the triangular or the carrier wave. So, this terms are used often frequently triangular wave or carrier wave, modulating wave or reference wave these terms are interchangeably used. So, in this case in the TS period time period we have the VAO waveform here shown like this and we can get what is the average VAO during this time period TS. what is the average value? So, for that we will do some very basic geometrical analysis. • From geometry, ?? ?? = ?? ?? • So, 1−? ?? 2 −??? = 1 ?? 2 • Thus, ? = ??? ?? 2 = duty ratio. • The average voltage of vAO during the switching cycle Ts is: ???(??) = ?? ??? ?? 2 + 0. ?? 2 −??? ?? 2 = ??? Now, this means VAO average is equal to VD into Ton by TS by 2 and this Ton divided by TS by 2 which we have derived earlier is equal to m. So, therefore, VAO average is m times VD which is also understood from this figure because if m is equal to 0 we do not get any average voltage, if m equal to 1 we get the full average voltage. So, if m is 0 the modulating waveform is down here, if m equal to 1 the modulating waveform is up here, ok. (Refer Slide Time: 36:55) So, VAO average is the average voltage in a switching cycle TS which we have just now derived. Now, if we start to vary the value of m slowly from cycle to cycle ok. In this particular cycle which we see here the value of m is constant over the switching cycle, but suppose in the next switching cycle we just change the value of the modulating waveform slightly. So, for example, which I have shown it here in this diagram, the value of the modulating waveform in the first switching cycle is here, in the second switching cycle it has been slightly increased the third switching cycle here it has been further increased slightly.
, if m is varying slightly from cycle to cycle then what do we expect, we expect that the average voltage will also vary from cycle to cycle, right. So, the average voltage will also change in each cycle. (Refer Slide Time: 38:23) So, if now we change the value of m sinusoidally over a period of time, then the average output voltage will also vary sinusoidally and this is how we generate a sinusoidal output from a converter. Say for example, I show you this diagram here, in this diagram we see that the reference waveform is changing sinusoidally, but it is changing sinusoidally very slowly as compared to the time period in of the triangular waveform. The triangular waveform frequency is much higher than the frequency of the sine wave. So, a small portion of the triangular waveform period, the sine wave it seems is not changing at all. So, it is more or less fixed. So, this is what we I had shown it here in this diagram. In the triangular period the modulating waveform is almost like fixed, it is not changing, because the frequency of the triangular wave is very very high compared to the modulating wave. And that is what I had shown in fact, I had zoomed a small window of this waveform here and I had shown in the previous slide, this slide here was a small window of this waveform which was the full waveform. So, if m varies sinusoidally and slowly over time then the VAO average will vary sinusoidally with time. Now, you may argue what do we mean by VAO average varying. So, sometimes in order to differentiate people have used the term instantaneous average voltage in this case. Instantaneous average voltage means over a time period TS the average voltage is m times VD here. So, this is the instantaneous average voltage whereas, months m starts to vary here then we get a sinusoidal output from the converter and this assumption is only valid if the triangular frequency is very high as compared to the modulating waveform or the reference waveform. For example, the modulating waveform can be a 50 Hertz or a 60 Hertz signal whereas, the triangular frequency can be 5 kilo Hertz or 10 kilo Hertz or even higher. So, during a small portion of the triangular period we can safely assume that the modulating waveform sinusoidal is fairly constant. Now, one important thing if m does not vary sinusoidally then so which, so now, we have come to this point when we say that the output voltage of the converter can be varied and that can be varied by using the modulating waveform as a sinusoidal waveform, if I use a non sinusoidal waveform can it be done? Type equation here.In fact, yes it can be done. (Refer Slide Time: 42:39) For example, suppose this is the triangular waveform and we are generating a sinusoidal waveform from the triangular wave, but the as far from our understanding the waveform need not to be sinusoidal. For example, if suppose we have a waveform like this and I want to generate something like this from the converter then it is also possible. So, the modulating waveform need not to be a sinusoidal waveform in fact, it can be a higher order harmonic waveform also.
The only condition that we have to preserve is that the frequency of the highest component in this non sinusoidal wave from which we are generating. The frequency of this the frequency the highest frequency component in this waveform must be very less compared to the frequency of the triangular waveform or in other words the frequency of the triangular waveform should be much higher than the highest frequency of the waveform that we want to generate; typically, 15 times higher, 10 to 15 times higher. So, this kind of a non sinusoidal waveform is used for example, in active filters where the converter is asked to produce a non-sinusoidal waveform containing different harmonics. And, in that case we must have a triangular waveform which is even higher than the highest frequency harmonics that the converter is producing. So, coming back to this concept of sinusoidal PWM, we see that by changing the modulating waveform slowly and sinusoidally over time we can generate a sinusoidal output voltage from the converter and this is nothing, but called sinusoidal PWM.
High Power Multilevel Converters – Analysis, Design and Operational Issues Dr. Anandarup Das Department of Electrical Engineering Indian Institute of Technology, Delhi Lecture – 03 Basic Understanding of Converter – (Sinusoidal pulse width modulation and three phase circuit) (Refer Slide Time: 00:17) So, in the half bridge, what is the expression of output voltage and it is given by this formula present here .
Now, this factor here V D by 2, this factor is because we have taken the reference point of the pole voltage as the lower side or negative pole of the DC bus. If we take the reference point for calculating the pole voltage as the midpoint or the imaginary midpoint of the DC bus, then this V D by 2 will cancel out and the AC output or the AC component inside the pole voltage is given by this expression here m sine omega t into V D by 2. So, the pole voltage or V AO has two parts; one is the AC part and one is the DC part. The DC part comes because of the choice of the reference point. If the choice of the reference is say it can be made in such a way that the DC part vanishes and then, we can say that V AO t is m sine omega t into V D by 2. Now, so, if we see here the AC component of the voltage, we find that it is proportional to m. Now, m is called the modulation index. So, m can vary, but usually it will vary from 0 to 1. Under some circumstances, it can go beyond 1. When it is varying between 0 and 1, we call it linear modulation. When it is more than 1, then we call it over modulation because if m is more than 1, then the sine wave, the height of the sine wave is more than the height of the triangular wave. Now, apart from this expression, here I have talked about the fundamental AC as well as the DC, but there are some other high frequency harmonics also generated and these harmonics we will cover later. (Refer Slide Time: 03:06) So, how does the waveform look like? See it is shown in this curve here. The blue curve here is the instantaneous pole voltage or that means, V AO t with respect to the negative of the DC bus and we can see that this V AO t is varying between 0 and 600, where V D is taken as 600 as I have shown it here, V D is 600 volts DC bus. This is typical for 400 volt, 415 volt, three-phase AC. So, the 600 volt DC bus, so the V AO t is varying between 0 and 600. The pink curve here, this curve pink curve is the AC which is embedded which is embedded inside this instantaneous PWM waveform. And why it is embedded? We can understand it intuitively by observing the width of the pulses.
As we can see the width of the pulse is narrow here and the width of the pulse is large here and progressively, it becomes narrow until it becomes very narrow here. So, of course, it means that the average voltage is going down here and then, it progressively increases as I can see here and it becomes maximum at this point. So, the AC is embedded inside this PWM waveform or the Pole Voltage Waveform. Here, as I have also written it here the fundamental output voltage of the converter is linearly proportional to m and this is very important. As I have shown it in the previous expression, the AC component in this waveform is m sine omega t into V D by 2. So, V D being a constant so, the output voltage is linearly proportional to m. So, this is the advantage of the linear modulation, linear sine triangle PWM or linear sinusoidal PWM that the output voltage can be controlled by varying ‘m’ and it is linearly proportional. So, if ‘m’ is equal to 0, the fundamental V AO peak of the fundamental voltage in V AO is equal to 0. If ‘m’ is equal to 1, then the peak of the fundamental voltage inside A O or the peak of the fundamental voltage in the pole is V D by 2. Because m equal to 1 means and at sine omega t equal to 1 that is omega t equal to 90 degree. This V AO fundamental peak is V D by 2. So, if m is equal to 1, the fundamental RMS voltage in V AO is V D by 2 root 2; 1 by root 2 of the previous value, 1 by root 2 of this value. So, V D by 2 root 2 and that will be equal to almost 0.35 V D. So, the output voltage of the pole voltage output from the half bridge converter can be controlled between 0 and V D by 2 root 2 or in the RMS and in the peak, it is 0 and V D by 2; between these 2 values linearly with the change of m. (Refer Slide Time: 07:11) What will happen if m goes beyond 1, which means that the AC voltage here is in more than 1 is something like this. What will happen if m is more than 1? So, if m is more than 1, then the fundamental voltage output fr
om V AO is more than V D by 2. So, we get more output voltage. However, this region is called the over modulation region, but in this case we have a drawback and a serious drawback and that is the over modulation generates many lower order harmonics fifth-seventh etcetera. So, it is generally avoided; low over modulation operation of a converter is usually avoided because lot of fifth, seventh and other lower order harmonics are generated from the converter. (Refer Slide Time: 08:21) If we go on increasing the value of m, even further at one point of time, the square wave operation of the converter is reached. So, what is meant by square wave operation? In square wave operation, as you can see here each switch of the half bridge switches once in the cycle. So, the top switch is switched for 50 percent of the cycle and the bottom switch is switched for rest of the 50 percent of the cycle. So, here you can see that the bottom switch is switching for half the cycle. Here, so, we get 0 voltage and for half the cycle the top switch is switched. So, that we get the 600 volt output. Here, 600 volt output. So, if we do the Fourier analysis of this waveform and if we ignore or take out the DC part, there is a and that if we take out the DC part out of this waveform, then the fundamental peak voltage which is inside this squared wave is ; as we see and which is equal to 1.27 times that obtained with sine PWM. So, because 4 by pi is 1.27. So, in sine PWM the maximum fundamental peak voltage that we got was V D by 2. While in square wave, the maximum fundamental we could get is 1.27= 4 by pi times V D by 2, that is 1.27 times that what I we can obtain from sine PWM. So, this region between 1 and 1.27 is part of over modulation. Later, we will see that it is possible by injection of triple n harmonics or third harmonic, it is possible to extend the linear modulation by 15 percent more. We will come to that later. But as of now we can understand that the peak fundamental
voltage that we can obtain from a half bridge converter with sinusoidal PWM is V D by 2 that is the maximum voltage peak. While in square wave, it is 1.27 times this value. Again with a square wave, we get the same problems as we get in over modulation that is a large number of lower order harmonics are generated. So, we usually avoid the square wave mode of operation of the converter, we usually avoid this operation. (Refer Slide Time: 11:31) Now, once we have understood the half bridge that is now turn our attention to the full bridge circuit. Full bridge is nothing but an extension of half bridge. That is as I had told you that there are 2 switches here, which is forming 1 leg of the converter and you can see V AO voltage is present here. In a full bridge, we introduce 1 more leg here and these 2 legs are independent of each other. So, they do not know about each other. So, leg B can be controlled fully independently of leg A. So, what is the benefit, if I use a full bridge suppose I connect my load between point A and point B. So, there are 2 legs; leg A and leg B and these are their 2 outputs, A and B and the load is connected here across AB. So, from one half bridge that is for example, from leg A, I can obtain the peak fundamental voltage as V D by 2 if I use sine PWM from 1 leg that is leg A. Now, how much voltage can be obtained from the full bridge? Now, this voltage which is applied across the load we can term it as V AB. Now, this voltage . So, I can write like V AB is equal to nothing but V AO minus V BO ok. So, t
herefore, the total voltage which can be obtained across A and B is nothing but the difference of voltage between what I obtained from A leg and the B leg. So, if now I produce a voltage where leg B, the voltage produced by leg B is 180 degree phase shifted from the voltage produced by leg A ok. If I do like that, then I we can get double the voltage from a full bridge converter ok. So, if the voltage of phase B is 180 degree from phase A. (Refer Slide Time: 14:38) So, here you can see this in this curve that suppose the voltage of A phase is this; the voltage of A phase the fundamental voltage inside A phase is this much, the voltage produced by B Phase B the fundamental component of it is shown by this blue curve.
- beginner and advanced students also